The lung-on-a-chip can mimic the physical and mechanical capabilities of a human lung.
Wyss Institute for Biologically Inspired Engineering, Harvard University/Flickr
Par Chengpeng Chen, University of Maryland, Baltimore County
Bringing a new drug to market costs billions of euros and can take more than ten years. These huge investments of money and time are major contributors to skyrocketing healthcare costs and are significant barriers to bringing new therapies to patients. One of the obstacles lies in the laboratory models used by scientists to develop drugs.
Preclinical trials, i.e. studies that test the efficacy and toxicity of a drug before it enters clinical trials in humans, are mainly carried out on cell cultures and animals. These two methods are limited by their poor ability to reproduce the conditions of the human body. Cell cultures in a petri dish are unable to replicate all aspects of tissue functioning, such as how cells interact in the body or the dynamics of living organs. And animals aren’t humans — even small genetic differences between species can be magnified into major physiological differences.
Less than 8% of successful animal studies for cancer therapies end up in human clinical trials. Because animal models often fail to predict drug effects in human clinical trials, these late failures can significantly increase costs and health risks for patients.
To solve this transposition problem, researchers have developed a promising model that can more closely mimic the human body: the organ-on-chip.
As an analytical chemist, I worked on the development of organ and tissue models that avoid the oversimplification of common cell cultures and the divergences of animal models with humans. I believe that with further development, organs on a chip can help researchers study disease and test drugs in more realistic conditions.
What are Organs on a Chip?
In the late 1990s, researchers found a way to layer elastic polymers to control and analyze fluids on a microscopic scale. Thus was born the field of microfluidics, which, in the field of biomedical sciences, involves the use of devices capable of mimicking the dynamic flow of fluids in the body, such as blood.
Advances in microfluidics have provided scientists with a platform to grow cells that function more like the human body, especially with organs on a chip. The “chip” refers to the microfluidic device that houses the cells. They are usually made using the same technology as computer chips.
Not only do organs on a chip mimic the blood flow in the body, but these platforms also feature microchambers that allow multiple cell types to be integrated to mimic the various cell types normally present in an organ. Fluid flow connects these types of cells, making it possible to study how they interact with each other.
This technology can overcome the limitations of static cell cultures and animal studies in several ways. First, the presence of circulating fluid in the model allows it to mimic both what a cell experiences in the body, such as how it receives nutrients and eliminates waste, and how a drug travels through the blood and interacts with several types of cells. The ability to control the flow of fluids also makes it possible to fine-tune the optimal dosage of a particular drug.
The lung-on-a-chip model, for example, is capable of integrating the mechanical and physical qualities of a living human lung. It is able to mimic the expansion and contraction, or inspiration and expiration, of the lung and simulate the interface between the lung and the air. The ability to reproduce these qualities makes it possible to better study lung deficiency as a function of different factors.
How to use this technology more?
Although organs on a chip are pushing the limits of upstream pharmaceutical research, this technology has not yet been widely integrated into drug development pipelines. I think one of the biggest barriers to wide adoption of these chips is their high complexity and low practicality.
Current organ-on-a-chip models are difficult to use. Additionally, since most models are single-use and allow only one input, which limits what researchers can study at any given time, their implementation is both expensive and time- and labor-intensive. workforce. The high investments required to use these models might dampen enthusiasm for their adoption. After all, researchers often use the least complex models available for preclinical studies to reduce time and cost.
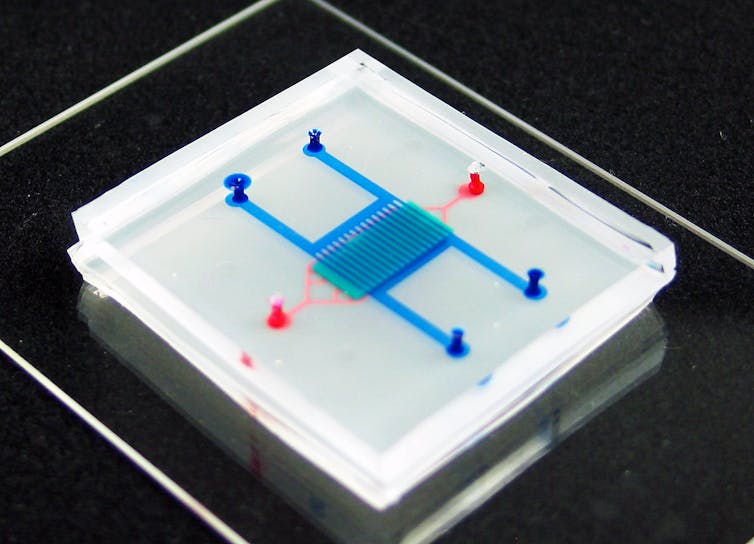
Vanderbilt University/Flickr
It is essential to lower the technical threshold for the manufacture and use of organs on a chip to allow the whole community to fully benefit from their advantages. But this does not necessarily mean simplifying the models. My lab, for example, has designed several ready-to-use, standardized and modular tissue chips, making it easy to assemble prefabricated parts to perform their experiments.
The advent of 3D printing has also significantly facilitated the development of organs on a chip, allowing researchers to fabricate entire models of tissues and organs directly on chips. 3D printing is ideal for rapid prototyping and design sharing among users and also facilitates the mass production of standardized materials.
I believe that organs on a chip have the potential to enable breakthroughs in drug discovery and better understanding of how organs function, whether healthy or diseased. By making this technology more accessible, we might take the model out of laboratory development and let it prevail in the biomedical industry.
Chengpeng Chen, Assistant Professor of Chemistry and Biochemistry, University of Maryland, Baltimore County
This article is republished from The Conversation under a Creative Commons license. Read the original article.