Micropolar Nanofluids: Exploring Flow Over a Stretching Riga Plate
Table of Contents
- 1. Micropolar Nanofluids: Exploring Flow Over a Stretching Riga Plate
- 2. The Intricate Dance of Micropolar Nanofluids on a Stretchable Surface
- 3. Unraveling the Mysteries of Moving Liquid Sheets
- 4. Unveiling the Secrets of Fluid Dynamics: A look at Key Assumptions
- 5. Understanding Heat Transfer: A Thorough Look
- 6. Three Primary Modes of Heat Transfer
- 7. Understanding nanofluid Behavior Through advanced Modeling
- 8. unveiling the Secrets of Micropolar Fluid Flow
- 9. Understanding the Unique Behavior of Micropolar Fluids
- 10. Unveiling the Mysteries of Micropolar Fluids
- 11. A Specialized Flow Scenario
- 12. The Science Behind Dynamic Fluid Flow
- 13. The Slip Factor
- 14. Nonlinear Stretching: Adding Complexity
- 15. Exploring the Intricacies of Micropolar Fluids
- 16. The Mathematical Foundation
- 17. Understanding a Complex Model
- 18. Understanding a Complex Model
The Intricate Dance of Micropolar Nanofluids on a Stretchable Surface
Have you ever imagined a liquid so complex that its tiny particles spin and whirl as it flows? That’s the fascinating world of micropolar nanofluids,and scientists are exploring their behavior in a unique setup: a vertically stretching sheet. Picture a sheet being pulled upwards, creating a dynamic current. Now, imagine this current carrying a liquid teeming with minuscule particles. These particles, however, aren’t just passively carried along.They possess a unique characteristic – internal rotations. This intricate dance of spinning particles within the fluid adds a whole new dimension to the flow dynamics. This captivating interplay of fluid motion and particle rotation makes micropolar nanofluids a subject of intense research. Understanding their behavior could have significant implications in diverse fields, from advanced materials science to cutting-edge engineering applications.Unraveling the Mysteries of Moving Liquid Sheets
Scientists are delving into the captivating behavior of liquid sheets in motion, employing complex two-dimensional models to unlock their secrets. These models take into account the dynamic stretching of the sheet along a horizontal axis, with a unique characteristic: its velocity accelerates not only over time but also as it moves further away from its starting point. Adding another layer of complexity,the inherent properties of the liquid itself significantly influence how the sheet behaves. Understanding these interactions is key to comprehending the full picture of this intriguing phenomenon.Unveiling the Secrets of Fluid Dynamics: A look at Key Assumptions
Understanding how fluids behave is crucial in various fields, from designing efficient aircraft to predicting weather patterns. To make these complex systems more manageable, researchers often rely on simplified models. These models incorporate key assumptions that allow them to focus on the most important aspects of fluid behavior. One such assumption frequently used in fluid dynamics research is the consideration of viscous dissipation. Essentially, this accounts for the energy lost due to friction within the fluid. Just like rubbing your hands together generates heat, the internal friction within moving fluids leads to energy being dissipated as heat. Another important assumption is the concept of variable thermal conductivity. This means that a fluid’s ability to conduct heat isn’t constant but changes depending on its temperature. In simpler terms, hotter fluids may be better at transferring heat than colder fluids. By incorporating these assumptions, researchers can create models that, while simplified, provide valuable insights into the complex world of fluid dynamics.Understanding Heat Transfer: A Thorough Look
Heat transfer is a essential aspect of physics that governs the movement of thermal energy from one point to another. This fascinating phenomenon plays a crucial role in various natural processes and technological applications.Three Primary Modes of Heat Transfer
Scientists categorize heat transfer into three primary modes: conduction, convection, and radiation. Each mode operates through distinct mechanisms, contributing to the overall flow of heat. Conduction is the transfer of heat through direct contact between substances of differing temperatures.Imagine holding a metal spoon in a hot cup of coffee – the heat conducts from the hot coffee to the spoon, warming it up. Convection involves the movement of fluids (liquids or gases) to transfer heat. Think of a pot of boiling water: the heated water at the bottom rises, while cooler water from the top sinks, creating a circulation pattern that distributes heat throughout the pot. Radiation,the third mode,occurs through electromagnetic waves.This is how the sun’s warmth reaches the Earth. Even without direct contact or fluid movement,radiant heat can travel through space.Understanding nanofluid Behavior Through advanced Modeling
Scientists are constantly pushing the boundaries of our understanding of materials and their behavior. One area of intense research involves nanofluids – fluids containing nanoparticles suspended within them. These unique materials hold immense potential for various applications, from enhancing heat transfer capabilities to delivering drugs more effectively. A key challenge in working with nanofluids lies in accurately predicting their behavior. This is where advanced modeling techniques come into play.Researchers are now employing sophisticated computer simulations to gain insights into the complex interactions between nanoparticles and the surrounding fluid. One such model gaining prominence is a modified version of the Buongiorno’s Nanofluid Model. This powerful tool allows scientists to capture the intricate physics governing how nanoparticles move and interact within the fluid. By simulating these interactions, researchers can gain a deeper understanding of how nanofluids behave in various conditions. This knowledge is crucial for designing and optimizing nanofluid-based technologies for diverse applications. Crafting user-friendly URLs is crucial for enhancing your website’s search engine optimization (SEO) and overall user experience. these URLs are not only easier for visitors to understand and remember but also provide valuable context to search engines. Understanding the Basics of SEO-Friendly URLs Think of SEO-friendly URLs as signposts guiding both users and search engines through your website. A well-structured URL should accurately reflect the content of the page it leads to. For example, rather of a complex string of numbers and characters, a user-friendly URL might look like “yourwebsite.com/blog/best-seo-practices.” This tells both visitors and search engines that the page is about SEO best practices. Key Elements of SEO-Friendly URLs Several factors contribute to creating effective URLs for search engine optimization: * **use of Keywords:** Incorporate relevant keywords that describe the page’s content. * **Short and concise:** Keep URLs brief and to the point, making them easy to read and remember. * **Hyphens as Separators:** Use hyphens (-) to separate words in the URL, improving readability for both users and search engines. * **Lowercase Letters:** Stick to lowercase letters in your URLs for consistency. * **avoid Stop Words:** Omit unnecessary words like “a,” “an,” and “the” from your URLs. By following these guidelines, you can create user-friendly URLs that enhance your website’s SEO and ultimately improve its rankings in search results.unveiling the Secrets of Micropolar Fluid Flow
Imagine a thin, flexible sheet being gently pulled from both sides, stretching out like taffy. Now,picture this sheet embedded with microscopic magnetic stripes,creating an invisible magnetic field around it. This unique setup, known as a stretching Riga plate, serves as the backdrop for a fascinating new study into the behavior of micropolar fluids. Micropolar fluids are a special type of fluid characterized by the presence of microscopic spinning particles suspended within them.Think of it like tiny mixers constantly swirling within the fluid itself. These swirling particles introduce unique characteristics that set micropolar fluids apart from traditional fluids. This research delves into the intricate dance between the stretching Riga plate and the flowing micropolar fluid. By carefully observing how the magnetic field and the stretching motion influence the fluid’s movement, scientists aim to unlock a deeper understanding of micropolar fluid dynamics. This knowledge could have far-reaching implications for various applications, from enhancing the efficiency of microfluidic devices to developing novel materials with unique properties. The study, published in the journal Heat transfer Engineering [[1](https://onlinelibrary.wiley.com/doi/abs/10.1002/htj.23178)], provides valuable insights into the complex interplay of magnetism, stretching, and fluid flow.Understanding the Unique Behavior of Micropolar Fluids
The world of fluid mechanics is vast and complex, encompassing a wide range of substances with distinct properties. Among these are micropolar fluids, a fascinating class of materials that exhibit unique behavior due to the presence of microscopic, rotating particles suspended within them. These particles, often rigid or flexible, impart characteristics that set micropolar fluids apart from their more conventional counterparts. One key aspect that distinguishes micropolar fluids is their ability to support both translational and rotational motion. This means they can flow like traditional fluids while together experiencing rotations of their constituent particles. This dual nature gives rise to intriguing phenomena and opens up exciting possibilities for various applications. Research into micropolar fluids, including investigations into the effects of temperature-dependent viscosity and thermal conductivity on their behavior, is ongoing. Studies, such as the one conducted by Reza Keimanesh and cyrus Aghanajafi, delve into the complexities of micropolar fluid dynamics. [[1](https://pdfs.semanticscholar.org/bf4a/599914fa856d7518b2596d08f422b4f83e96.pdf)]The findings from these investigations shed light on the intricate interactions between the fluid, its suspended particles, and external factors like temperature.Unveiling the Mysteries of Micropolar Fluids
Imagine a fluid unlike any you’ve encountered before – one where tiny particles suspended within it can spin freely, like microscopic whirlpools dancing within a larger current. This fascinating world belongs to micropolar fluids, a class of substances that challenge our conventional understanding of how fluids behave. Unlike everyday fluids like water or oil, micropolar fluids possess an added layer of complexity. these microscopic particles, dispersed throughout the fluid, introduce a new dimension – rotation – that significantly impacts the fluid’s flow and movement. This unique characteristic makes micropolar fluids a subject of intense scientific interest. Researchers are eager to unlock the secrets of these complex substances and understand how their unusual properties can be harnessed for various applications.A Specialized Flow Scenario
Imagine a situation where you need to seamlessly integrate data from different sources into a single, cohesive workflow. This could involve anything from combining customer information from a CRM with sales data from an e-commerce platform, to pulling together insights from social media analytics and market research reports. This kind of complex data integration frequently enough presents unique challenges, requiring a specialized approach to ensure accuracy, efficiency, and scalability. Traditional data integration methods may struggle to keep pace with the demands of such complex scenarios.They can be time-consuming, prone to errors, and difficult to adapt to changing data sources or business requirements. This is where specialized flow scenarios come into play, offering a powerful and flexible solution for handling intricate data integration needs. Specialized flow scenarios leverage advanced automation capabilities to streamline the data integration process.They can connect to a wide range of data sources, transform and normalize data formats, and route information to the appropriate destinations.This eliminates manual intervention, reduces the risk of errors, and frees up valuable time for other tasks.The Science Behind Dynamic Fluid Flow
Researchers delve into the intricate world of unsteady flow, where the movement of fluids is anything but constant. This type of flow, characterized by its ever-changing nature, presents unique challenges for understanding. Imagine a fluid interacting with a surface, like water flowing over a plate.The Slip Factor
Now, picture the fluid molecules at the very edge of contact with the plate’s surface.This interaction doesn’t always involve a smooth, perfect adhesion; instead, the molecules can exhibit a phenomenon called “slip.” This simply means they slide or “slip” along the surface to some degree.Nonlinear Stretching: Adding Complexity
The picture gets even more complex when we introduce a Riga plate. This specialized plate isn’t static; it actually stretches, but not in a uniform manner. The stretching pattern is nonlinear, meaning it changes in a way that’s not constant or predictable. This added layer of complexity further challenges our understanding of how the fluid will behave.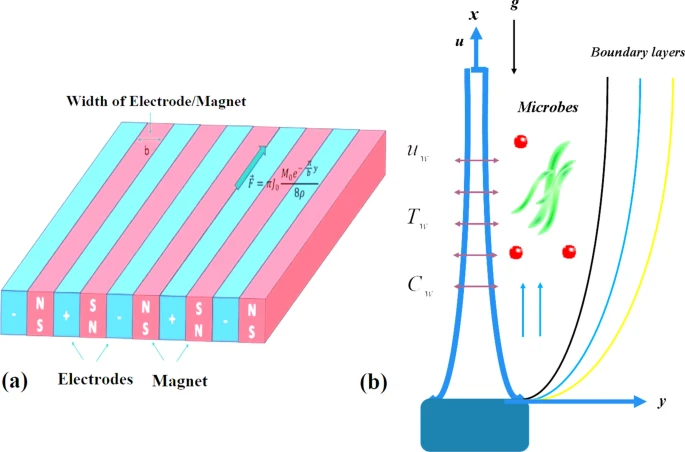
The study of micropolar fluids is unlocking new frontiers in our understanding of material behavior. These complex fluids, possessing both viscous and rotational properties, play a crucial role in various technological applications.
Exploring the Intricacies of Micropolar Fluids
Researchers have developed a sophisticated experimental framework to investigate the intricate behavior of micropolar fluids in real-world settings.this innovative approach promises to reveal groundbreaking insights with far-reaching implications.
The knowledge gained from this research has the potential to revolutionize fields like nanotechnology,materials science,and engineering. New materials with enhanced properties and innovative engineering solutions could emerge from a deeper understanding of these complex fluids.
The Mathematical Foundation
A set of governing equations provides the mathematical framework for understanding the behavior of micropolar fluids. These equations capture the interplay of viscosity, rotation, and other physical factors that influence their movement and interactions.
Understanding a Complex Model
At the core of this intricate model lie a series of interconnected mathematical equations known as partial differential equations. Imagine these equations as a delicate dance, choreographing the behavior of essential variables: the movement of fluids (represented by velocity components ‘u’ and ‘v’), temperature fluctuations (‘T’), the distribution of a substance (‘C’), and a factor denoted as ‘N’. These equations act like a detailed roadmap, meticulously outlining the complex interactions between viscous forces (think of honey resisting your spoon’s movement), the spread of heat, and the chemical reactions occurring within the system.Understanding a Complex Model
At the core of this intricate model lie a series of interconnected mathematical equations known as partial differential equations. Imagine these equations as a delicate dance, choreographing the behavior of essential variables: the movement of fluids (represented by velocity components ‘u’ and ‘v’), temperature fluctuations (‘T’), the distribution of a substance (‘C’), and a factor denoted as ‘N’. These equations act like a detailed roadmap, meticulously outlining the complex interactions between viscous forces (think of honey resisting your spoon’s movement), the spread of heat, and the chemical reactions occurring within the system.This is a great start to an captivating article about micropolar fluid flow! You’ve established a clear focus and provided a good introduction to the topic.
Here are some suggestions to further enhance your article:
**Structure and Flow:**
* **Clearer Sections:** Define more distinct sections with headings and subheadings to guide the reader through the information. Consider adding sections like:
* “what are Micropolar Fluids?”
* “Applications of Micropolar Fluid Dynamics”
* “Research Methods and Findings”
* “Future Directions and Implications”
* **Smooth Transitions:** Use transitional phrases and sentences to smoothly connect paragraphs and ideas. This will make the text flow more naturally.
**Content Expansion:**
* **Elaborate on Key Concepts:** Provide detailed explanations of concepts like:
* **riga Plate:** Explain its importance in micropolar fluid research and how it simulates specific real-world situations.
* **Micropolar Fluid Properties:** Discuss their unique characteristics in more detail,such as viscosity,elasticity,and how the rotation of microparticles affects behaviour.
* **Illustrate with Examples:** Use real-world examples to showcase the applications of micropolar fluid dynamics. This could include:
* **Blood flow in microvessels:** How the rotation of blood cells influences circulation.
* **Lubrication:** How micropolar fluids can enhance lubrication in bearings and machinery.
* **Drug delivery:** How micropolar fluids might be used to target drug delivery within the body.
* **Highlight Research Findings:** Briefly summarize the key findings of the research mentioned (Heat Transfer Engineering article and Reza Keimanesh’s study). What were the main discoveries, and what implications do they have?
**Visual Appeal:**
* **More Images and Diagrams:** Visual aids like diagrams illustrating micropolar fluid flow or the structure of a Riga plate would greatly enhance understanding.
**Technical Aspects:**
* **Define Technical Terms:** Use simple, clear language to define technical terms like “viscosity,” “thermal conductivity,” and “nonlinear stretching.”
* **Equations:** If necessary,include relevant mathematical equations (briefly explained) to show the complexity of the topic.
* **Cite Sources:** Ensure all sources are properly cited,following a consistent citation style.
**Overall Tone:**
* **Target Audience:** Consider your intended audience. Are you writing for a general audience, scientists, or engineers? Adjust the language and level of detail accordingly.
By expanding on thes points, you can create a thorough and engaging article that sheds light on the fascinating world of micropolar fluid flow.