Well, well, well! Gather ’round, my lovely nerds, and let’s dive into the whimsical world of digital PCR! Now, before you start thinking this is just about a fancy computer game, let me enlighten you.
Digital PCR, or dPCR if you’re trying to impress your mates at the lab, is the lovechild of polymerase chain reaction (PCR) and a tiny party of little reactions happening all at once. First introduced back in the late ’90s by the dynamic duo, Vogelstein and Kinzler, it has morphed from a somewhat clunky, complex method to the belle of the laboratory ball. Honestly, it’s like the transformation of a caterpillar to a butterfly—just with a lot more DNA involved!
We’re talking about a technique that allows you to actually count the number of target molecules in your sample. Yes, folks! You can quantify stuff without getting bogged down by standard curves, which—let’s be real—sounds about as exciting as watching paint dry. Instead of guessing, you know what you’ve got! It’s like going fruit shopping and coming home with just bananas instead of a mystery fruit salad.
Now, the commercial aspect has been booming too! If you thought juice cleanses were all the rage, think again. The digital PCR market is projected to balloon from a modest $584.5 million in 2023 to a jaw-dropping $3.679 billion by 2032. Someone get me a slice of that cake!
In the UK, the Genetics Core at the Edinburgh Clinical Research Facility is leading the charge with dPCR. Dr. Tamara Gilchrist, who sounds like she has superhero-level lab skills, explained that this platform is all about precision—offering the ability to identify rare DNA alleles as easily as I can identify a bad pun.
But like every good romance, there are challenges too. Designing effective primers and probes is no walk in the park, especially if you’re multiplexing—yes, I said multiplexing! It’s like trying to juggle when you already struggle to keep one ball in the air. The process can be so intricate that it could put hairdressers to shame with all the processing precision.
Let’s not forget the elephant in the room—or should I say the expensive equipment in the lab? dPCR systems tend to require some serious cash, which may not be available everywhere. This isn’t your average DIY science project!
Now, for all you budding scientists out there wondering why you’d pick dPCR over other PCR methods, it’s simple: sensitivity and precision. You don’t want to be that person at the party overestimating your cocktail measurements, do you?
In the clinical arena, digital PCR shines like a diamond in the rough. It can detect circulating tumor DNA in cancer patients non-invasively—how’s that for taking a blood sample without a side of extreme discomfort? And it’s becoming more popular for tracking disease progression.
For those looking to keep things smooth in the lab, Tammy offers some sage advice: optimize those primers and avoid contamination like it’s a bad date. Her golden rule? Keep good documentation. Yes, I hear the groans, but trust me, you’ll thank me later!
So, there you have it. Digital PCR is where science meets commodities, bringing sensitivity and precision without the fuzzy math. Whether you’re in the lab or just keen to show off your smarts, this technique is set to revolutionize the way we quantify DNA. And who knows, maybe it’ll even inspire a sci-fi movie one day! Now, if only it could help me quantify the number of times I’ve been asked, “Isn’t science boring?” Spoiler alert: it’s not!
Digital PCR, a sophisticated form of polymerase chain reaction (PCR), was initially introduced to the scientific community by renowned researchers Vogelstein and Kinzler in the late 1990s. Prior to this landmark study, various adaptations of digital PCR methodologies existed, yet they were typically categorized under the umbrella of “limiting dilution PCR.”
While digital PCR originally presented challenges for laboratories due to its complexity and the extensive time and labor it demanded, the evolution of technology has ushered in a new era. The emergence of novel platforms that streamline digital PCR processes has facilitated its adoption across numerous laboratories, especially those involved in cutting-edge clinical research. According to market research data, the global digital PCR market stood at an impressive $584.5 million in 2023, with projections suggesting a phenomenal surge to $3,679 million by 2032.
The Genetics Core at the Edinburgh Clinical Research Facility exemplifies a UK-based laboratory that has embraced digital PCR, consistently employing this technology in their research endeavors. The Core is dedicated to providing secure procedures for receiving, processing, archiving, and analyzing biological samples, thereby supporting the comprehensive pipeline of clinical research—from initial sample collection to in-depth genetic analysis.
Dr. Tamara (Tammy) Gilchrist plays an integral role as a research technician within the Genetics Core. In her interview with Technology Networks, Dr. Gilchrist offers a detailed exploration into digital PCR, revealing its advantages over alternative PCR methodologies and sharing invaluable insights for successful experimentation.
Molly Coddington (MC):
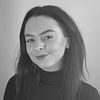
Senior Writer and Newsroom Team Lead
Technology Networks
Molly Coddington is a Senior Writer and Newsroom Team Lead at Technology Networks. She holds a first-class honors degree in neuroscience. In 2021, Molly was shortlisted for the Women in Journalism Georgina Henry Award.
Can you explain what digital PCR is, and discuss its evolution as a technique?
Tamara Louise Gilchrist, PhD (TLG):
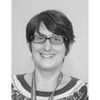
Tamara (Tammy) Louise Gilchrist is a research technician in the Edinburgh Clinical Research Facility Genetics Core, where she has worked for the last 10 years. Her role is currently focused on next-generation sequencing and she also is the lead for digital PCR. Previously, Tammy was a research assistant in the Bone Research group at the University of Edinburgh.
Digital PCR functions remarkably similarly to quantitative real-time PCR (qPCR); however, it allows absolute quantification of target molecules within a sample by compartmentalizing one large PCR reaction into thousands of tiny individual reactions. This can be achieved using various formats such as microwells, channels, or oil droplets, depending on the technology employed.
We utilize a microfluidic platform capable of generating up to 30,000 individual droplets, or “crystals,” on a self-contained consumable chip. As interest in digital PCR has surged, the methodologies for dispersal have also evolved, aimed at enhancing both the quantity and the fidelity of these partitions.
Similar to qPCR, each micro-reaction in digital PCR generates a fluorescent signal when the target is present through the use of TaqMan chemistry or intercalating dyes. However, in digital PCR, the detection occurs at the endpoint, identifying micro-reactions as positive or negative. Positive droplets indicate the presence of one or more copies of the target molecule present within the sample. System software relies on established statistical models based on Poisson distribution to deduce the absolute amount of the target within the sample by assessing the count of positive versus negative responses.
To ensure precise calculations, equal sample volume must be maintained across partitions, as larger volumes would naturally yield higher target copy numbers, hence compromising Poisson distribution comparability. Furthermore, quantification uncertainty can be mitigated by escalating the number of analyzed partitions, fostering a reduction in potential calculation errors.
Due to differences in dispersal methods and fluorescent signal detection, specific platforms may be more suitable for particular experimental applications, which should be carefully considered when designing experiments.
With ongoing advancements, digital PCR technology continues to evolve, allowing for the simultaneous detection of multiple targets from a single sample. Today, various multiplexing strategies have been developed, significantly amplifying the capabilities of digital PCR.
MC:
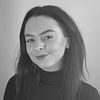
Senior Writer and Newsroom Team Lead
Technology Networks
Molly Coddington is a Senior Writer and Newsroom Team Lead at Technology Networks. She holds a first-class honors degree in neuroscience. In 2021, Molly was shortlisted for the Women in Journalism Georgina Henry Award.
Why might a researcher choose to conduct digital PCR over other types of PCR?
TLG:
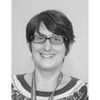
Tamara (Tammy) Louise Gilchrist is a research technician in the Edinburgh Clinical Research Facility Genetics Core, where she has worked for the last 10 years. Her role is currently focused on next-generation sequencing and she also is the lead for digital PCR. Previously, Tammy was a research assistant in the Bone Research group at the University of Edinburgh.
One of the primary motivations for utilizing digital PCR revolves around its exceptional precision and sensitivity. Unlike other methods, digital PCR negates the need for a standard curve, allowing a direct calculation of concentration instead of relying on extrapolated correlations to known standards, effectively enhancing precision and reproducibility compared to traditional qPCR.
The compartmentalization of samples into numerous separate reactions boosts the likelihood of detecting elusive alleles or targets that exist in extremely low quantities. This compartmentalization also tolerates a higher level of impurities that may act as PCR inhibitors since potential contaminants become diluted within the small sample volumes.
Many digital PCR systems further impress researchers with the ability to multiplex, allowing for simultaneous detection of multiple targets from a limited volume of sample material. This ability is particularly crucial for scenarios where precious or scarce samples are involved, thereby significantly augmenting the potential outputs of experiments.
MC:
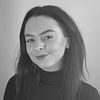
Senior Writer and Newsroom Team Lead
Technology Networks
Molly Coddington is a Senior Writer and Newsroom Team Lead at Technology Networks. She holds a first-class honors degree in neuroscience. In 2021, Molly was shortlisted for the Women in Journalism Georgina Henry Award.
Are there any limitations or challenges associated with digital PCR?
TLG:
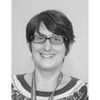
Tamara (Tammy) Louise Gilchrist is a research technician in the Edinburgh Clinical Research Facility Genetics Core, where she has worked for the last 10 years. Her role is currently focused on next-generation sequencing and she also is the lead for digital PCR. Previously, Tammy was a research assistant in the Bone Research group at the University of Edinburgh.
One of the primary obstacles faced in digital PCR is the nuanced design of effective primers and probes, especially in multiplexing scenarios. While multiplexing presents a tantalizing opportunity to detect multiple targets in a single experimental run, it necessitates extensive assay development—a process often arduous due to the precision required in designing components that prevent cross-reactivity and confirm reliable detection of unique targets.
To address these challenges, several companies are launching new solutions featuring standardized universal fluorescent reporters, along with custom assay options that can greatly simplify the development phase, thereby making it less intimidating for researchers.
Additionally, the requirement for specific and often expensive capital equipment and consumables presents another barrier to entry that may limit accessibility for certain labs.
By offering digital PCR services in our genomics core facility, we aim to make both equipment and the associated expertise readily available, fostering greater accessibility for researchers across various backgrounds and career stages.
MC:
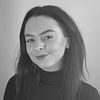
Senior Writer and Newsroom Team Lead
Technology Networks
Molly Coddington is a Senior Writer and Newsroom Team Lead at Technology Networks. She holds a first-class honors degree in neuroscience. In 2021, Molly was shortlisted for the Women in Journalism Georgina Henry Award.
What are the most common clinical research applications of digital PCR technology?
TLG:
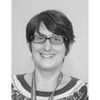
Tamara (Tammy) Louise Gilchrist is a research technician in the Edinburgh Clinical Research Facility Genetics Core, where she has worked for the last 10 years. Her role is currently focused on next-generation sequencing and she also is the lead for digital PCR. Previously, Tammy was a research assistant in the Bone Research group at the University of Edinburgh.
Our laboratory predominantly employs digital PCR to detect DNA released into the bloodstream, such as circulating tumor DNA (ctDNA) from patients with cancer or identifying biomarkers indicative of inflammation in conditions like inflammatory bowel disease.
Being able to non-invasively profile tumors using digital PCR to analyze ctDNA offers significant advantages. This technology is instrumental for identifying therapy-resistant mutations and monitoring disease progression, even when targets exist in minute quantities that might be overshadowed by background DNA.
Additionally, we are exploring the integration of digital PCR into our next-generation sequencing (NGS) workflows, as it enhances the accuracy of NGS libraries quantification, improving sample pooling precision. However, the balance between enhanced quantification accuracy and the cost implications compared to conventional methodologies remains under evaluation.
MC:
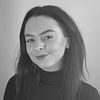
Senior Writer and Newsroom Team Lead
Technology Networks
Molly Coddington is a Senior Writer and Newsroom Team Lead at Technology Networks. She holds a first-class honors degree in neuroscience. In 2021, Molly was shortlisted for the Women in Journalism Georgina Henry Award.
What advice do you have for running a successful digital PCR experiment?
TLG:
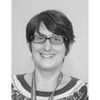
Tamara (Tammy) Louise Gilchrist is a research technician in the Edinburgh Clinical Research Facility Genetics Core, where she has worked for the last 10 years. Her role is currently focused on next-generation sequencing and she also is the lead for digital PCR. Previously, Tammy was a research assistant in the Bone Research group at the University of Edinburgh.
The cornerstone of a successful digital PCR experiment lies in meticulous experimental design tailored to the specific research questions being addressed. It’s imperative to carefully choose the platform, as each platform presents unique benefits as well as inherent limitations. Reflecting on my experiences, I’ve found that the execution of the digital PCR process itself is relatively straightforward.
Prioritizing the optimization of primers and probes pays dividends long-term, particularly in multiplexing conditions to prevent cross-reactivity or quenching phenomena.
Due to the assay’s sensitivity, avoiding contamination is key, which necessitates using dedicated pipettes, filter-equipped tips, and working in a clean environment to minimize contamination risks. Ensuring that all equipment is correctly calibrated and well-maintained is crucial. Including both positive and negative controls in every run strengthens the reliability of the results.
Thorough documentation is critical to experimental integrity. The Digital MIQE Guidelines (Minimum Information for Publication of Quantitative Digital PCR Experiments) delineate a standardized protocol for key information that must be gathered and reported in digital PCR studies. Consulting these guidelines during the experimental design phase significantly enhances the quality and reproducibility of digital PCR data.
P class=”MsoNormal”>Additionally, utilizing appropriate sample preparation techniques is crucial, as the quality of the sample directly impacts the accuracy and sensitivity of the assay results. Running control samples alongside experimental samples can help validate the efficiency of the digital PCR reactions.
data analysis is a critical step that should not be overlooked. Employing robust statistical methods to interpret digital PCR results can enhance the reliability of the conclusions drawn from the experiments.